Quantum computing is no longer a theoretical concept confined to academic research and science fiction; it is becoming a technological reality that promises to revolutionize industries, economies, and entire fields of study. While traditional computers operate using bits that represent data as 0s or 1s, quantum computers utilize quantum bits, or qubits, which can represent and process far more complex information. This leap in computational capability opens the door to solving problems that are currently intractable for even the most powerful supercomputers.
In this article, we will explore the emerging role of quantum computing, its potential applications across various industries, the challenges that must be overcome, and the profound ways in which it may shape the future of technology. We will also delve into how quantum computing differs from classical computing, the current state of the field, and the potential timeline for mainstream adoption.
What is Quantum Computing?
To understand quantum computing’s impact, we first need to grasp the basic concepts that differentiate it from classical computing. In traditional computing, data is processed in binary form: bits, which are strictly 0 or 1. Every computation is a series of operations performed on these bits. While classical computers have become exponentially more powerful over time, they are fundamentally limited in how they can handle extremely complex problems due to the binary nature of their processing.
Quantum computing, on the other hand, operates based on the principles of quantum mechanics, the fundamental theory in physics that describes nature at the smallest scales of energy levels of atoms and subatomic particles. The two key principles that enable quantum computing’s power are superposition and entanglement.
- Superposition
In quantum computing, qubits can exist in a state of superposition, meaning that a qubit can represent both 0 and 1 simultaneously, rather than just one or the other. This ability to hold multiple states at once dramatically increases the computational power of quantum systems. As the number of qubits in a quantum computer increases, the system’s ability to perform complex computations grows exponentially. - Entanglement
Quantum entanglement is a phenomenon where two or more qubits become interconnected, such that the state of one qubit directly influences the state of another, regardless of the distance between them. This property allows quantum computers to process information in ways that classical systems cannot, by leveraging the instantaneous correlation between entangled qubits to solve intricate problems much faster.
These principles enable quantum computers to perform many calculations simultaneously, making them ideal for solving highly complex problems in fields such as cryptography, material science, and artificial intelligence—tasks that would take classical computers an impractical amount of time to compute.
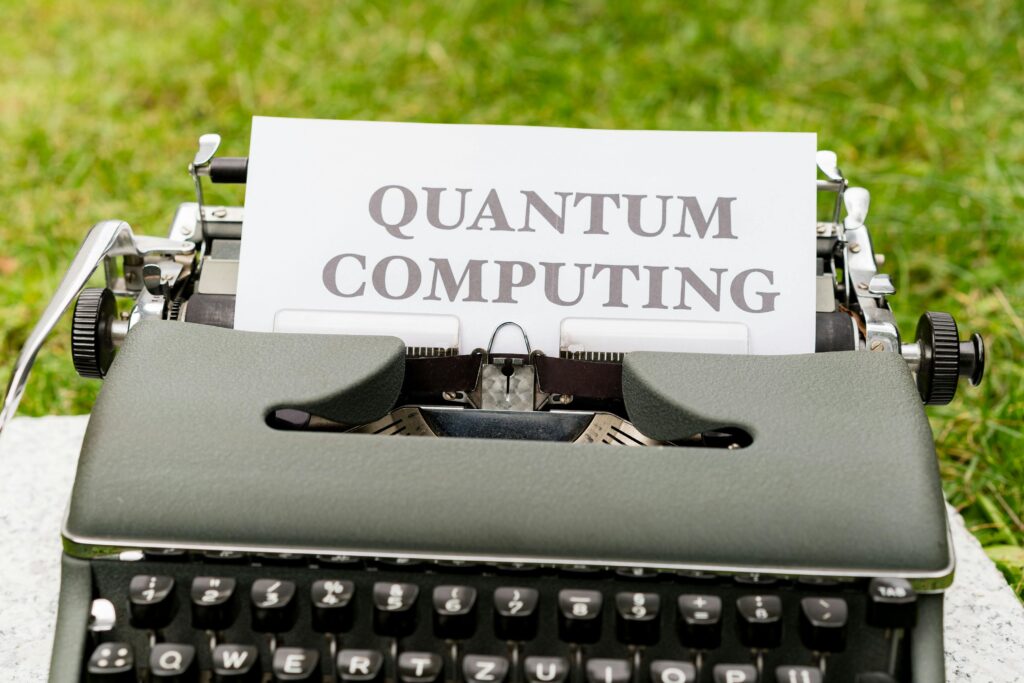
How Quantum Computing Differs from Classical Computing
While both classical and quantum computers aim to solve problems, their approaches are radically different:
- Binary vs. Quantum States: Classical computers rely on bits that are either 0 or 1. Quantum computers, using qubits, can exist in multiple states simultaneously due to superposition, vastly increasing computational potential.
- Computation Power: The power of classical computers scales linearly with the number of bits, while quantum computers’ power scales exponentially with the number of qubits. For example, a quantum computer with just a few hundred qubits could outperform a classical supercomputer in specific tasks.
- Parallelism: Classical computers perform one operation at a time in sequence, whereas quantum computers can perform multiple operations simultaneously thanks to superposition and entanglement.
Quantum computers do not aim to replace classical computers but rather complement them, providing solutions to complex problems that are beyond the reach of traditional systems.
Quantum Computing: Current State and Challenges
Although the potential of quantum computing is immense, it is still in its early stages of development. There are numerous technical challenges to overcome before we can achieve practical, large-scale quantum computing.
- Quantum Decoherence
One of the biggest challenges facing quantum computing is quantum decoherence, which occurs when qubits lose their quantum properties due to interference from their environment. This results in errors during computation, as the fragile quantum states of superposition and entanglement are difficult to maintain for extended periods. Scientists are working to develop error-correcting algorithms and stable qubit environments to mitigate this issue. - Qubit Stability (Noise)
Quantum systems are highly sensitive to environmental noise, such as temperature fluctuations and electromagnetic interference. This instability causes qubits to lose their quantum state, making computations inaccurate. Maintaining a controlled and stable environment for qubits, often requiring cryogenic temperatures, is one of the significant hurdles in quantum computing development. - Error Rates and Fault Tolerance
Due to their fragile nature, qubits are prone to errors during computations. Quantum error correction is an area of active research, aiming to create fault-tolerant quantum systems that can maintain computational accuracy even when errors occur. Current quantum computers are still far from achieving the low error rates required for large-scale, reliable quantum processing. - Scalability
Building quantum computers with a large number of qubits is another major challenge. Current quantum computers typically operate with a few dozen qubits, but to solve complex real-world problems, systems with thousands or even millions of qubits will be required. Developing scalable quantum architectures is one of the primary goals of researchers in the field.
Despite these challenges, significant progress has been made in recent years. Major technology companies like IBM, Google, and Microsoft, as well as startups such as Rigetti Computing and IonQ, are actively developing quantum hardware and software platforms. In 2019, Google’s quantum computer, Sycamore, achieved a milestone known as quantum supremacy—the point at which a quantum computer can solve a problem that would be practically impossible for classical computers to solve within a reasonable timeframe. This achievement marked an important step toward realizing the potential of quantum computing.
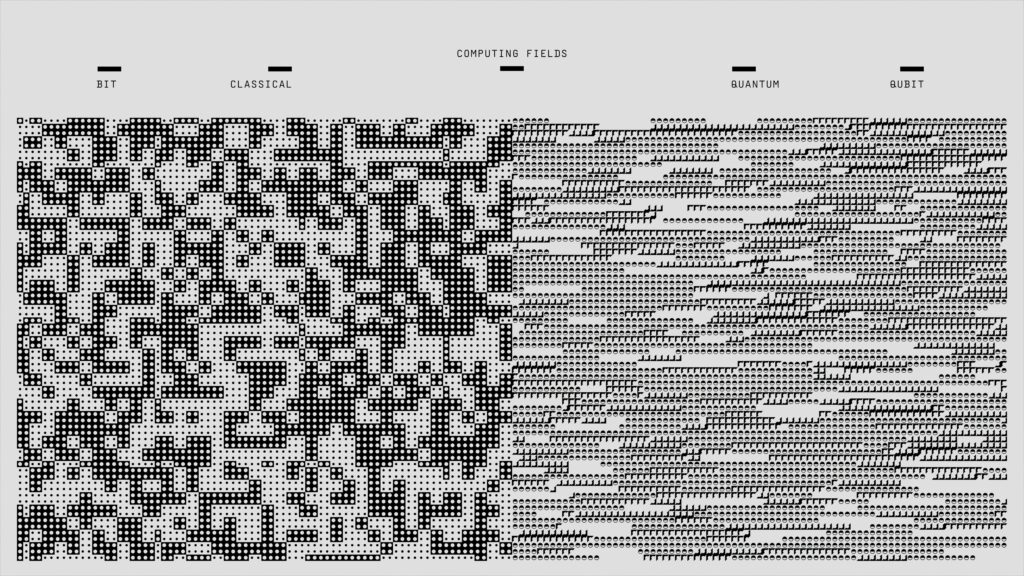
Quantum Computing Applications: Industries Poised for Transformation
Quantum computing has the potential to revolutionize many industries by solving problems that are beyond the reach of classical computers. Here are some key industries where quantum computing is expected to have the most significant impact:
- Cryptography and Cybersecurity
Quantum computing will have a profound effect on the field of cryptography. Most modern encryption techniques, such as RSA and elliptic-curve cryptography, rely on the difficulty of factoring large numbers or solving complex mathematical problems, tasks that classical computers struggle to accomplish efficiently. However, Shor’s algorithm, a quantum algorithm, could potentially break these encryption methods by efficiently factoring large integers.
This poses a significant risk to current cryptographic protocols, prompting governments and businesses to explore quantum-safe cryptography, which would be resistant to attacks by quantum computers. While this creates a challenge for cybersecurity, quantum computing also offers solutions. Quantum key distribution (QKD), for example, leverages quantum mechanics to create secure communication channels that are theoretically immune to eavesdropping. - Drug Discovery and Healthcare
Quantum computing’s ability to simulate molecular interactions at the quantum level will be transformative for drug discovery and healthcare. Classical computers struggle to simulate the behavior of molecules due to the immense complexity involved in their quantum mechanical interactions. Quantum computers, however, can efficiently model these interactions, allowing researchers to understand molecular properties better and develop new drugs faster.
For instance, quantum simulations could help discover new materials and compounds that target specific diseases, dramatically accelerating the drug development process. Additionally, personalized medicine could benefit from quantum computing by analyzing genetic information at an unprecedented scale, enabling tailored treatment plans for individuals based on their genetic makeup. - Materials Science
Quantum computers have the potential to revolutionize materials science by simulating the properties of new materials and substances at the quantum level. Today, scientists rely on approximations and experimental data to understand the properties of new materials, which can be time-consuming and costly. With quantum computing, researchers could simulate material properties more accurately and quickly, leading to breakthroughs in the development of high-performance materials for industries such as aerospace, energy, and construction.
For example, quantum simulations could help design more efficient batteries, superconductors, and photovoltaic cells, contributing to the development of more sustainable and energy-efficient technologies. - Artificial Intelligence and Machine Learning
Quantum computing could significantly accelerate the development of artificial intelligence (AI) and machine learning algorithms. Classical computers often struggle to process the vast amounts of data required for training complex AI models. Quantum computers, with their ability to perform parallel computations, could handle these tasks more efficiently.
Quantum machine learning (QML) is an emerging field that explores how quantum algorithms can improve machine learning processes. For example, quantum computers could enhance pattern recognition and optimization tasks, leading to faster and more accurate AI models. This could revolutionize industries ranging from finance to healthcare by enabling more powerful predictive models and real-time decision-making systems. - Finance and Risk Management
The financial industry is another area poised to benefit from quantum computing. Financial institutions rely heavily on complex algorithms to assess risk, optimize portfolios, and forecast market trends. Quantum computing can improve these processes by providing faster and more accurate calculations.
For example, Monte Carlo simulations, which are used to model the probability of different outcomes in financial systems, could be executed more efficiently on quantum computers. This would enable better risk management strategies and more accurate pricing models for financial instruments. Additionally, quantum computing could optimize trading strategies by solving complex optimization problems in real-time, giving traders a competitive edge in fast-moving markets. - Logistics and Supply Chain Management
Logistics companies often deal with complex optimization problems, such as determining the most efficient routes for delivery trucks or minimizing supply chain costs. These problems are known as combinatorial optimization problems, where classical computers struggle to find the optimal solution due to the sheer number of possible combinations.
Quantum computing can address these challenges by solving optimization problems more efficiently. This could result in more efficient supply chains, reduced transportation costs, and faster delivery times, benefiting industries from e-commerce to manufacturing. - Climate Modeling and Environmental Science
Climate change is one of the most pressing challenges facing humanity, and quantum computing could play a crucial role in addressing this issue. Climate models are incredibly complex, involving countless variables and interactions that classical computers struggle to simulate accurately. Quantum computers could help create more accurate models of climate systems, enabling researchers to better understand and predict the effects of climate change.
Additionally, quantum simulations could aid in the development of new technologies for reducing greenhouse gas emissions, such as more efficient carbon capture systems or cleaner energy sources.
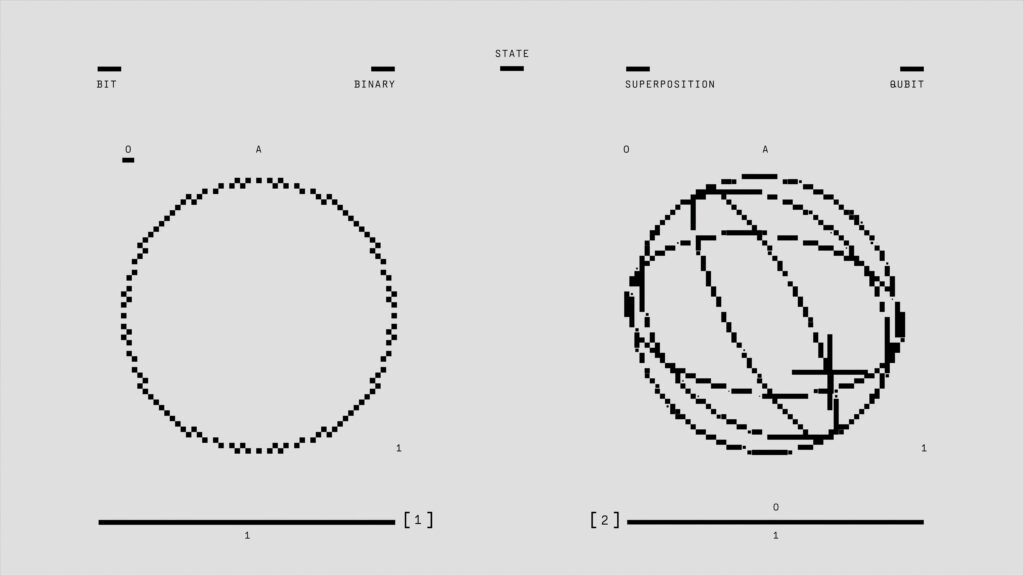
The Road to Quantum Computing Adoption: Challenges Ahead
While quantum computing has the potential to revolutionize industries, widespread adoption is still several years away. There are several key challenges that must be addressed before quantum computers become practical for everyday use:
- Hardware Development
Quantum computing hardware is still in its infancy, with most quantum computers currently limited to tens of qubits. To solve real-world problems, we will need quantum computers with thousands or millions of qubits, as well as more stable qubits that can maintain their quantum states for longer periods. Researchers are exploring different approaches to building scalable quantum computers, including superconducting qubits, trapped ions, and topological qubits. - Software and Algorithms
Developing software and algorithms that can take full advantage of quantum computing is another significant challenge. Quantum computers operate fundamentally differently from classical computers, requiring new programming paradigms and algorithms. While researchers have developed quantum algorithms for specific tasks, such as Shor’s algorithm for factoring integers and Grover’s algorithm for searching unsorted databases, there is still much work to be done to create a comprehensive set of quantum tools for practical applications. - Integration with Classical Systems
Quantum computers are unlikely to replace classical computers entirely; rather, they will complement classical systems by solving specific types of problems. This means that businesses will need to develop hybrid computing environments that combine classical and quantum computing capabilities. Integrating these two systems seamlessly will require new frameworks, software, and infrastructure. - Cost and Accessibility
Quantum computers are currently expensive to build and maintain, requiring specialized environments such as cryogenic cooling systems. As the technology matures, costs are expected to decrease, but making quantum computing accessible to businesses and researchers will require significant investment in infrastructure and cloud-based quantum platforms.
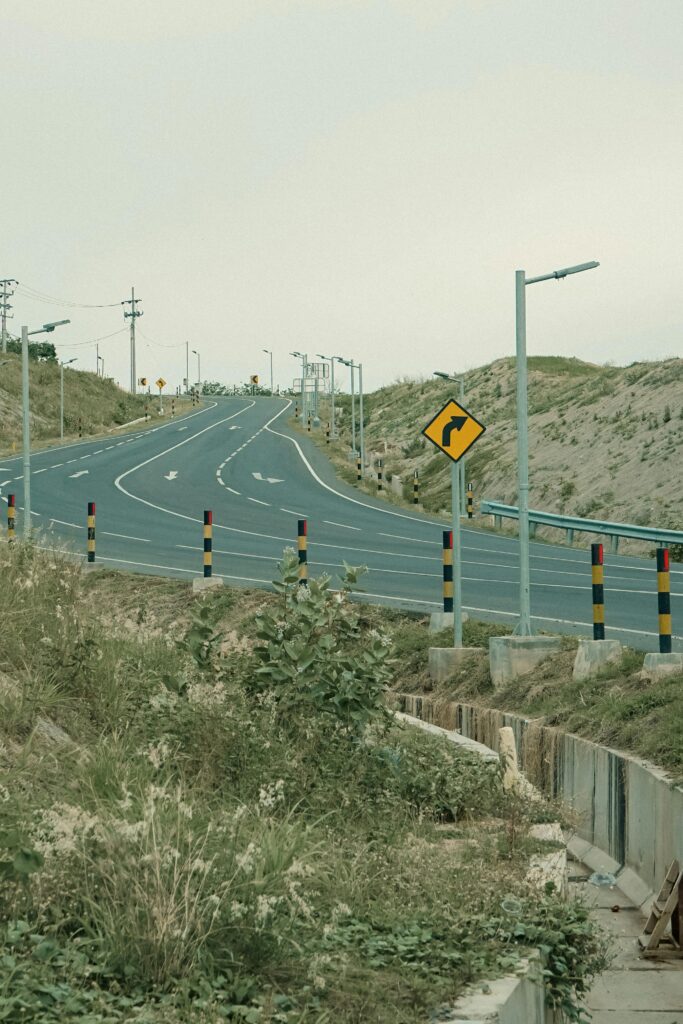
Conclusion: The Quantum Revolution is Just Beginning
Quantum computing is on the verge of transforming industries and reshaping the future of technology. With its unparalleled computational power, quantum computing has the potential to solve complex problems that are currently beyond the capabilities of classical computers, from cryptography to drug discovery, materials science, and artificial intelligence.
However, there are still significant challenges to overcome before quantum computing becomes a mainstream technology. Researchers must continue to develop more stable qubits, scalable quantum architectures, and efficient algorithms. While widespread adoption may still be years away, the progress made in recent years suggests that the quantum revolution is just beginning.
As industries prepare for the arrival of practical quantum computing, businesses and governments alike must invest in research, infrastructure, and workforce development to stay competitive in this new era of computing. Those who embrace quantum computing early will be well-positioned to capitalize on the immense opportunities it presents, driving innovation and creating new possibilities for solving some of the world’s most pressing challenges.
In the coming decades, quantum computing will undoubtedly reshape the technological landscape, and its role in advancing scientific discovery, improving cybersecurity, and tackling global challenges like climate change cannot be overstated. We are standing on the precipice of a new era, where the unimaginable becomes possible, and quantum computing will lead the way.